The main aim of this analysis is to study the effect of bonding strength between metal and ceramic at different processing temperature and holding time. The Al/SiC bonding was modelled and analyzed using finite element analysis in ANSYS software. The different values of coefficients of linear thermal expansion (CTEs) of the metal and ceramic are induces more thermal stress at the interface. The mismatch of thermal stress at the interface region plays an important role in improving bonding strength. Hence, it is essential to study and evaluate the interface bonding in metal-ceramics joints.
Keywords |
Bonding strength, Coefficient of thermal
expansion, Thermal stress, Interface |
INTRODUCTION |
The growing interest in aluminium based metal matrix
composites could be realized for the past decades because
of its superior mechanical and physical properties. The
mechanical properties are generally depending on the
interface bonding between matrix and reinforcement. It is
well known that the function of the interface is to transmit
load from matrix to reinforcement due to the large stress
gathering capability of the reinforcement and binding
property of the matrix material [1]. The formation of
interface bond was mainly affected by the factors such as
processing temperature and holding time. The different
processing temperature and holding time leads to thermal
expansion in Al and SiC. Due to their difference in
thermal expansion coefficient (CTEs) residual thermal
stress will be induced at the interface [2]. This thermal
stress will affect the interface bonding between Al/SiC,
which is known as thermal mismatch stress. Metalceramic
interfacial phenomena controls stress transfer
between Al/SiC [5]. |
Generally composite materials with weak interfaces
have relatively low strength and stiffness but high
resistance to fracture, whereas materials with strong
interfaces have high strength and stiffness but are
somewhat brittle [6, 7]. S. Sozhamannan et al, (2009),
studies the influence of processing temperature at constant
holding time in the interface bonding of Al/SiC. The
interfacial bonding strength increases with increase in
processing temperature due to least formation of
interfacial compounds. The structural morphologies of
interface in Al/SiC interface region. The higher
concentration of Si in the matrix region near the interface
alters the interface bonding characteristics of Al/SiC. The
diffusion rate of Si depends on the functions of
temperature and time. The objective of this paper is to
analyse the interface residual stress at different holding
time and temperature. |
FINITE ELEMENT MODELING |
Three-dimensional rectangular model of Al/SiC
were created in FEA to find the global and local stress and
strain status. Al/SiC FEA model shown in Fig.1. The
model adopted assumes that the SiC plate glued to
aluminium, while the matrix deform elastically or elasticplastically
depending on the local effective stress
level..The one end of contour was fixed, that is degrees of
freedom at all direction x, y and z are arrested. The
negative pressures were applied at other end of contour
which showed in below diagram. The finite element
modelling procedures are mentioned below in fig. 2. and
material properties of Al/SiC are given in table 1 |
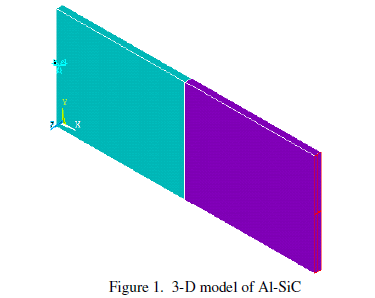 |
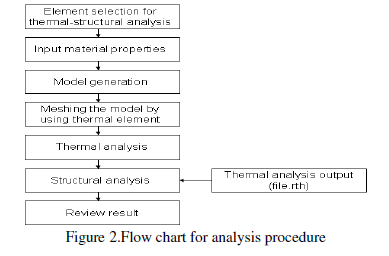 |
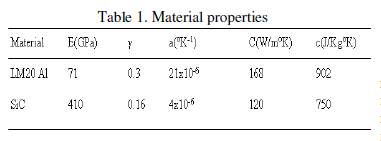 |
FINITE ELEMENT MODELING OF AL-SIC AND
LINE PATH DIAGRAM |
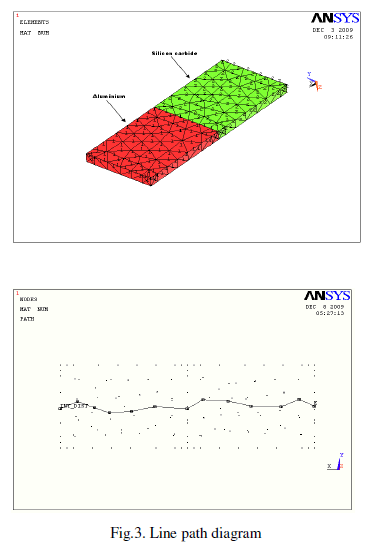 |
THERMAL STRESS DISTRIBUTION |
The thermal stress distribution across the interface
region is shows in fig.4 to fig.6 revealed that the
maximum thermal stress were present at the interface
region. It is clear evident that the thermal stresses at the
interface region increases when increasing processing
temperature with holding time. At lower temperature, the
thermal stress are low in the range from (-69.717 to -
169.93 MPa). At higher temperature (>800ºC),more
thermal stress were accumulated in interface region due to
the different thermal expansion value between SiC and Al
alloy, and also SiC thermo-dynamically unstable. The
presence of higher thermal stress may lead to debonding
at the interface and it reduce the load transferring across
the interface. |
THERMAL STRESS DISTRIBUTION DIAGRAM |
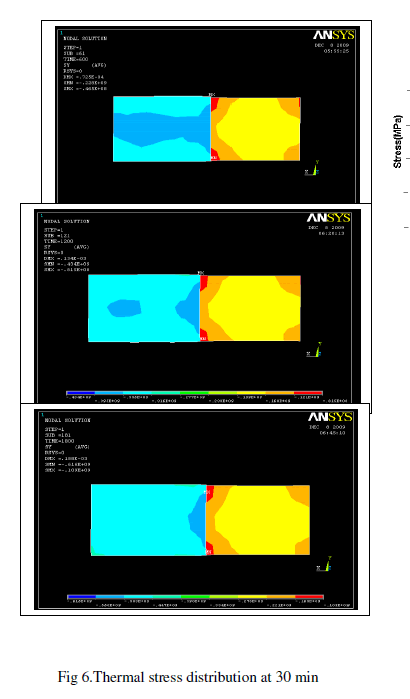 |
THERMAL STRESS DISTRIBUTION
BETWEEN Al-SiC (X-DIRECTION) |
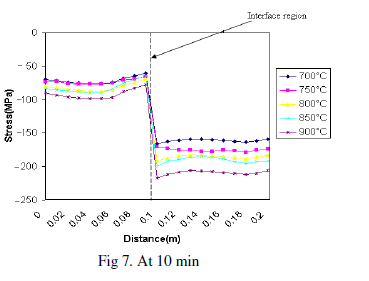 |
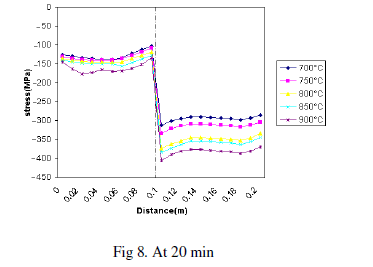 |
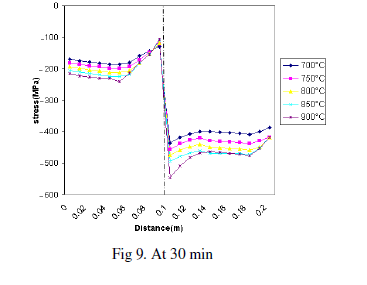 |
THERMAL STRAIN DISTRIBUTION
DIAGRAM |
The thermal strain characteristics
are plotted in fig: 10to 12. The strain distribution shows
that a maximum thermal strain has been found in the Al
region and also the strain distributions are very low in the
SiC side. There is a strain relaxed by the SiC are
transferred to the matrix, which results a matrix are
fractured as thermal strain increases. It was found that
thermal strains are increased when increase in processing
temperature and holding time between Al matrix and SiC
reinforcement. The presence of high strain at the interface
region cause the interface bond strength. Since the matrix
(Al) and reinforcement (SiC) have different thermal and
mechanical properties, they exhibit different thermal
strain distributions along the interface region. |
THERMAL STRAIN BETWEEN Al-SiC
(X-DIRECTION) |
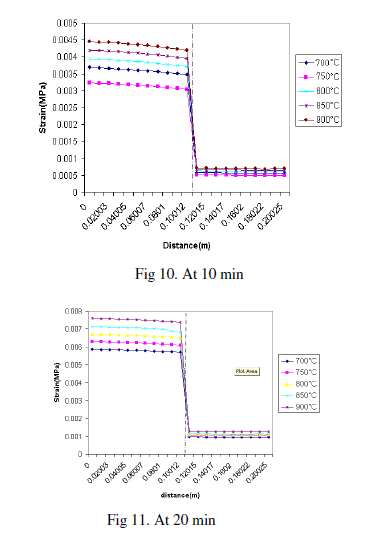 |
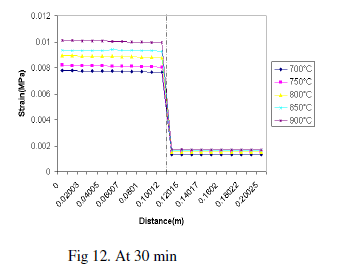 |
THERMAL STRESS DISTRIBUTION AT
INTERFACE REGION (Y-DIRECTION) |
The plot shows the thermal stress distribution
along the interface region (y-direction).From this plot ,it
shows the higher thermal stress induced at the corner of
the interface region and this phenomena gradually
increase with change in temperature and time. The
compressive thermal stress act on this interface region
between Al and SiC. Increase in thermal stress at corner
of the interface region induce thermal strain at the
reinforcement (SiC) side. There is a strain relaxed by the
SiC are transferred to the matrix which results a matrix
are fractured as thermal strain increases. |
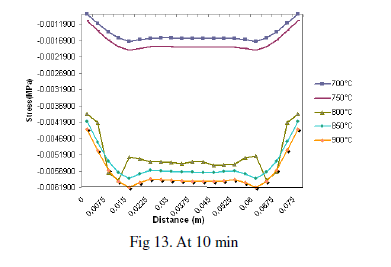 |
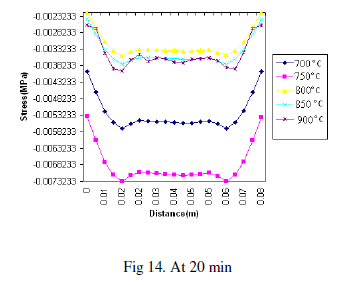 |
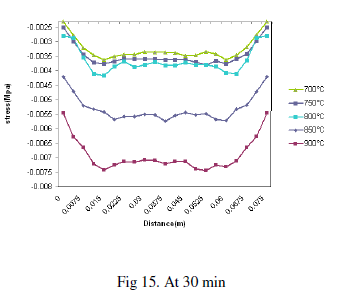 |
CONCLUSIONS |
• Increase in various processing temperature with
holding time, thermal expansion between Al and SiC
were increased. This phenomena increase thermal
stress at the interface region. |
• The higher thermal stresses induce more residual
stress at the interface region. This may leads to create
debonding between Al and SiC. |
• Increase in thermal stress will leads to increase
thermal strain at the interface region between Al and
SiC. The higher thermal strain cause interface
bonding strength between Al-SiC. |
• In the interface region, increases in temperature with
time, thermal stress were induced at the corner (top
and bottom) side. |
• The presence of higher thermal stress along the
interface leads to induce thermal strain in that region,
which results matrix fractured as thermal strain
increases |
References |
- G.G.Sozhamannan, S.BalasivanandhaPrabu, Influence ofinterface compounds on interface bonding characteristics ofaluminium and silicon carbide, Materials Characterization, vol 51,pp.1044-5803, 2009.
- S.BalasivanandhaPrabu, .Karunamoorthy.G.S. Kandasamy, AFinite Element analysis study of micromechanical interfacialcharacteristics of metal matrix composites ,Journal of MaterialsProcessing Technology, 153-154, pp .992-997, 2004.
- A. Bendada, A. Derdouri, M. Lamontagne, Y. Simard , Analysisof thermal contact resistance between polymer and mold ininjection molding, Applied Thermal Engineering, vol 24,pp .2029–2040, 2004.
- Vishal Singha, Anthony F. Black, Suresh V. Garimella, Anexperimentally validated thermo-mechanical model for theprediction of thermal contact conductance, Applied ThermalEngineering, vol 48, pp .5446–5459, 2005.
- F. Lau a, W.B. Lee , S.M. Xiong , B.C. Liu , A study of theinterfacial heat transfer between an iron casting and a metallicmould, Journal of Materials Processing Technology 79 25–29,1998.
- Yongyi Zhu, Thermal Contact Development, Applied ThermalEngineering, vol677, pp .1654-1232, 1999.
- A.M. Khounsary, D. Chojnowski, and L. Assoufid, ThermalContact resistance across a copper-silicon interface, Advancedphoton source, vol.604, pp . 60439-4843, 1997.
- A.M.S. Hamouda and M.S.J. Hashrni, Mechanical properties ofaluminium metal matrix composites under impact loading,Journal of Materials Processing Technology 56 pp. 743-756,1996.
- M. Kemal Apalak, RecepGunes, On non-linear thermal stressesin an adhesively bonded single lap joint, Computers and Structures vol 80, pp.85–98, 2002.
|