Keywords
|
SEPIC converter, Power Factor Correction, Harmonic reduction, PWM, PSIM. |
INTRODUCTION
|
Power conversion is converting electric energy from one form to another, converting between AC and DC or just varying the voltage and frequency or some combination of these. The power conversion systems can be classified according to the type of input and output power |
AC to DC (Rectifier) |
DC to AC (inverter) |
DC to DC (DC to DC converter) |
AC to AC (AC to AC converter) |
DC to DC converters are important in portable electronic devices such as cellular phones and laptop computers, which are supplied by power from batteries mainly. The majority DC to DC converters also control the output voltage. AC to DC converters (rectifier) is an electrical device that converts alternating current (AC), which periodically reverses direction, to direct current (DC), which flows in only one direction. The process is well-known as rectification. Rectifiers have several uses, however are often found serving as components of DC power supplies and high-voltage direct current power transmission systems. Rectification may well serve in roles other than to generate direct current for use as a source of power. Because of the alternating nature of the input AC sine waves, the method of rectification only produces a DC current that, while unidirectional, consists of pulses of current. Several applications of rectifiers, such as power supplies for television, radio and computer equipment, necessitate a steady constant DC current (as would be produced by a battery).Normally AC-DC conversion is carried out by simply rectifying the AC input and the rectified output voltage is filtered by means of a large valued capacitance to get a nearly constant DC output voltage. In this conversion, the input AC supply current is drawn in narrow pulses since the capacitor voltage value is nearly constant. This narrow pulse current of high peak, results in power quality problems to nearby consumers, which include higher value of THD on supply current, higher THD of input supply voltage, lower value of power factor and displacement factor and poor distortion factor. These large harmonic currents are undesirable because they not only produce distortion of AC line voltage but also result in conducted and radiated electromagnetic interference (EMI). The problem becomes more serious particularly when several drive units are connected to single phase supply where the input power pulsates at twice the frequency. Recent international regulations governing the power quality and harmonic currents pollution limits at the utility have placed an increased emphasis on the application of improved power quality AC-DC converters to feed the load. For ideal sine wave line voltage, harmonic currents do not contribute to active power, this result in increased value of RMS current and therefore produces higher losses in the utility line. It is a prime concern to consider these power quality issues to design an AC-DC power converter, which provides unity power factor at input mains and also results in close regulations of output DC voltage. The single-ended primary-inductance converter (SEPIC) is a DC/DC-converter topology that provides a positive regulated output voltage from an input voltage that varies from above to below the output voltage. This kind of conversion is helpful when the designer uses voltages (e.g., 12 V) from an unregulated input power supply such as a low-cost wall wart. The SEPIC converter topology is one that fulfils all above requirements without inverting output voltage polarity. The circuit was first developed at AT&T Bell laboratories in the mid 1970s and has not been very widely used until recently. Now it is becoming more and more popular, especially in power conversion systems where the input voltage varies in wide range. In typical DC-DC power conversion system the output voltage must be kept constant regardless of changes in the input voltage or in effective load resistance. Therefore, such system invariably requires feedback control loop. Its design is based on the dynamic model of DC-DC power conversion system. Several topologies of single phase AC-DC converters are implemented in buck, boost, and buck-boost converters with improving the power quality in terms of reducing the harmonics of input current [2]. In a buck type DC-DC converter reported in [1] & [3], the DC output voltage is controlled using the digital multi loop PID controller and high frequency-link(HFL) power conversion systems(PCSs). We have proposed a circuit to realize isolated buck boost DC to DC conversion; and the full-bridge (FB) boost converter, [4] in this circuit the input voltage is close to output voltage. The modified SEPIC converter is integrated with solar powered system [5]. The typical model used closed loop control with maximum power point tracking (MPPT) algorithm to get high efficiency. Studies and analysis of cuk converter circuit is controlled the duty ratio of the switching element with output constant was reported in [6]. In this paper introduce a buck converter and SEPIC converter working in a step-down mode of operation and using for the optimized equation [7]. |
The figure 1 shows the block diagram of single phase AC-DC SEPIC converter. In that, ac voltage is given as input and the diode rectifier converts the ac voltage into dc voltage. The converted dc voltage is given to SEPIC converter circuit and then the required output voltage is obtained.AC-DC converters produce steady direct current (DC) from alternating current (AC) inputs. In a typical converter, the AC input is rectified and connected to a high frequency, high voltage switching circuit employing a transformer to create the desired DC output voltage. |
Such DC-DC power conversion subsystems can be realized by different circuit topologies. Among them the buck, boost, buck-boost and Cuk converters are the basic and the most used. Each of the circuit topology has its advantages and disadvantages and the choice depends on requirements for power conversion system. In general, circuits with the switch referenced to the ground node are preferred to simplify the switch driving circuits. Additionally, the nonpulsating input current is desirable to minimize EMI and reduce the need for additional filter elements. Significant advantage of the power conversion system is also the ability to generate output voltage either above or below the input voltage. In Figure 2 the circuit topology of the non isolated SEPIC converter is presented. It consists of two inductances, two capacitors, one MOSFET transistor, one diode, the input voltage source and the load resistance at the output of the converter. The amount of energy exchanged is controlled through switch S1, which is classically a transistor such as a MOSFET. |
ANALYSIS OF THE SEPIC CONVERTER
|
A SEPIC is a type of DC-DC converter allowing the electrical potential (voltage) at its output to be less than, greater than, or equal to that at its input; the output of the SEPIC is controlled by the duty cycle of the control transistor. SEPIC is effectively a boost converter followed by a buck-boost converter, consequently it is like to a conventional buck-boost converter, other than has advantages of having non-inverted output (the output has the same voltage polarity as the input), passing through a series capacitor to couple energy from the input to the output (and thus can respond more gracefully to a short-circuit output), and being able of factual shutdown: after the switch S1 is turned off, its output drops to 0 V, following a rather hefty transient abandon of charge. SEPICs are helpful in applications in which a battery voltage can be above and below that of the regulator's intended output |
The schematic diagram for a basic SEPIC is shown in Figure 3 As with other switched mode power supplies (specifically DC-to-DC converters), the SEPIC interactions energy between the capacitors and inductors in order to convert from one voltage to another. The amount of energy exchanged is proscribed in switch S1, which is classically a transistor such as a MOSFET. MOSFET offers much higher input impedance and lower voltage drop than bipolar junction transistors (BJTs), and do not require biasing resistors as MOSFET switching is controlled by differences in voltage rather than a current, like with BJTs. |
A. CONTINUOUS MODE |
A SEPIC is said to be in continuous-conduction mode if the current through the inductor L1 never falls to zero. During a SEPIC's steady-state process, the average voltage crosswise capacitor C1 (VC1) is equal to the input voltage (VIN). Because capacitor C1 blocks direct current (DC), the average current across it (IC1) is zero, making inductor L2 the simply source of load current. Hence, the average current through inductor L2 (IL2) is the same as the average load current and hence independent of the input voltage .Looking at average voltages, the following can be written: |
(1) |
Because the average voltage of VC1 is equal to VIN, VL1 = −VL2. For this reason, the two inductors can be wound on the similar core. Since the voltages are the equivalent in magnitude, their belongings of the mutual inductance will be zero, assuming the polarity of the windings is correct. Also, since the voltages are the same into magnitude, the ripple currents from the two inductors will be equal in magnitude. The average currents are summed as follows: |
(2) |
When switch S1 is turned on is shown in Figure 4, current IL1 increases and the current IL2 increases in the negative direction. The energy to enhance the current IL1 comes from the input supply. Since S1 is a little while closed, and the instant voltage VC1 is just about VIN, the voltage VL2 is approximately −VIN. Therefore, the capacitor C1 supplies the energy to increase the magnitude of the current in IL2 and thus increase the energy stored into L2. The easiest method to visualize this is to consider the bias voltages of the circuit in a D.C. state, and then close S1.When switch S1 is turned off, the current IC1 becomes the similar as the current IL1, while inductors do not allow instantaneous changes during current. The current IL2 will keep on in the negative direction; within reality it never reverses direction. It can be seen from the figure that a negative IL2 will add to the current IL1 to increase the current delivered toward the load. Using Kirchhoff's Current Law, it can be shown that ID1 = IC1 - IL2. It can then be concluded, that whilst S1 is off shown in Figure 5, power is delivered to the load from both L2 and L1. C1, though is being charged by L1 throughout this off cycle, and will in turn recharge L2 throughout the on cycle. |
Because the potential (voltage) across capacitor C1 may reverse direction each cycle, a non-polarized capacitor should be used. Conversely, a polarized tantalum or electrolytic capacitor may be used in some cases, because the potential (voltage) across capacitor C1 will not change unless the switch is closed long enough for a half cycle of resonance among inductor L2, and by this time the current in inductor L1 could be quite large. The capacitor CIN is required to reduce the effects of the parasitic inductance and internal resistance of the power supply. The boost or buck capabilities of the SEPIC are possible because of capacitor C1 and inductor L2. Inductor L1 moreover switch S1 create a standard boost converter, which generates a voltage (VS1) that is higher than VIN, whose magnitude is determined by the duty cycle of the switch S1. Since the average voltage across C1 is VIN, the output voltage (VO) is VS1 - VIN. If VS1 is less than double VIN, after that the output voltage will be a lesser amount of than the input voltage. If VS1 is greater than twice VIN, after that the output voltage will be greater than the input voltage. The development of switched-power supplies can be seen by coupling the two inductors in a SEPIC converter together, which begins to resemble a Flyback converter, the most basic of the transformer-isolated SMPS topologies. |
B. DISCONTINUOUS MODE |
A SEPIC is said to be here discontinuous-conduction mode if the current through the inductor L1 is allowed to fall to zero. |
POWER QUALITY IMPROVEMENT TECHNIQUES
|
There are some control techniques to improve the power quality in the control circuits, which are voltage follower approach and average current control technique. These techniques are explained detail in below. |
A. VOLTAGE FOLLOWER APPROACH |
Figure.6 shows the block diagram of a single-phase AC-DC converter with voltage follower operation. As shown in Figure.6, voltage follower uses a very simple control feedback, which only requires output voltage sensing. The bridge rectifier is used at the input AC side with a power factor corrector using an inductor and capacitor combination. Now, a small value of output voltage, compared to the reference value and resulting value, passes through the output voltage controller, which generates the PWM output and is used for switching the converter. It has inherent power factor correction characteristics with constant duty ratio and switching frequency, offering an attractive solution for lower power applications. The output voltage regulation is provided by the feedback loop as shown in Figure.6 where the output sensed voltage is compared with a reference value and the error is amplified in a proportional integral (PI) controller which is compared with a saw-tooth ramp, thus providing the pulse to power switch. Therefore, this circuit is controlled by the difference in the on- time interval and the constant switching frequency fs. Advantages |
? Constant switching frequency |
? No need of current sensing |
? Simple PWM control |
B. AVERAGE CURRENT CONTROL TECHNIQUE |
Another control method, which allows a better input current waveform is the regular current control represented in Figure.7. Here the inductor current is sensed and filtered by a current error amplifier whose output drives a PWM modulator. In this way the inside current loop tends to minimize the error between the average input current and its reference. This later is obtained in the similar way as in the peak current control. The converter works in CICM, thus the same considerations done with regard to the peak current control can be applied. |
Advantages |
? Constant switching frequency. |
? No need of compensation ramp. |
? Control is fewer sensitive to commutation noises, due to current filtering. |
? Improved input current waveforms than for the peak current control since, near the zero crossing of the line voltage, the duty cycle is close to one, so reducing the dead angle in the input current. |
DESINGN OF PROPOSED SEPIC CONVERTER
|
The design steps for the proposed are as follows, |
Step 1: Switching Frequency |
Step 2: Duty Cycle |
(3) |
(4) |
Dmin, Dmax are the minimum and maximum duty cycle |
Step 3: Inductor Current |
(5) |
Step 4: Inductor L1 and L2 |
(6) |
Step 5: Output Ripple Voltage |
(7) |
Step 6: Output Capacitor |
(8) |
Step 7: Load Resistance |
(9) |
Based on the above steps, the design parameters are calculated as shown in Table I |
SIMULATION AND RESULTS
|
A. SIMULATED CIRCUIT DIAGRAM AND RESULTS FOR OPEN LOOP CONTROL OF SINGLE PHASE SEPIC CONVERTER |
Figure 8 shows the simulated diagram for an open loop control of single phase SEPIC converter. The switch is triggered at a switching frequency of 10 kHz. Both the THD and power factor are measured at the input side. Figure 9 and 10 shows the input voltage and current waveforms for the above circuit. The peak input voltage is measured as 18.849 V. Figure 11 and Figure 12 shows the output voltage and output current for the proposed converter.. Result analysis for open loop control of SEPIC converter is shown in Table 2. |
B. SIMULATED CIRCUIT DIAGRAM AND RESULTS FOR CLOSED LOOP CONTROL OF SINGLE PHASE SEPIC CONVERTER USING VOLTAGE FOLLOWER APPROACH |
Figure 13 shows the closed loop control of single phase SEPIC converter using voltage follower approach, in which the output voltage of the converter will be sensed and compared with the reference voltage, and then the error voltage is amplified in a PI controller. In PWM modulator, the error voltage is compared with the saw tooth ramp thus the pulse will be generated and given to the switch. |
The input voltage and the current waveforms for the above circuit are shown in figure 14 and 15. Figure 16 and Figure 17 shows the output voltage and output current waveforms for the closed loop control of SEPIC converter. Result analysis for the voltage follower approach of the SEPIC converter is shown in Table 2. |
C. SIMULATED CIRCUIT DIAGRAM AND RESULTS FOR CLOSED LOOP CONTROL OF SINGLE PHASE SEPIC CONVERTER USING AVERAGE CURRENT CONTROL TECHNIQUE |
The simulated diagram of SEPIC converter using average current control technique is shown in Figure 18. In this technique, as mentioned above, in this method both the voltage and current will be sensed. The rectified voltage from the diode bridge and the output voltage of the error amplifier are multiplied and it gives the reference current. This is called the outer loop i.e., the voltage loop. In the inner loop i.e., the current loop, inductor current is compared with the saw tooth ramp. The output pulse generated will be given to the switch. The input voltage and the current waveforms for the above circuit are shown in figure 19 and 20. |
Figure 21 and 22 shows the output voltage and output current waveforms for the closed loop control of SEPIC converter. Result analysis for the voltage follower approach of the SEPIC converter is shown in Table II. |
Figure 23 and 24 shows the THD and Power Factor chart for various control techniques. |
CONCLUSION
|
The design, simulation and development of single-switch Buck-Boost SEPIC converter with high frequency nonisolation has been carried out for 15v output. With this designed converter, simulation has been done in standard PSIM (Power Simulation) software. High power quality is obtained with design parameters with power factor in the order of 0.97 and THD in the order of 1.12% using the Power Quality Improvement Techniques. Simulated and test results on the developed converter show the improved performance of the proposed high frequency Non-isolated AC-DC SEPIC converter in terms of low THD of supply current and improved power factor of AC mains. |
Tables at a glance
|
 |
 |
Table 1 |
Table 2 |
|
|
Figures at a glance
|
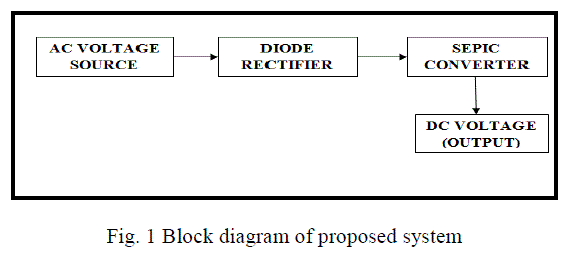 |
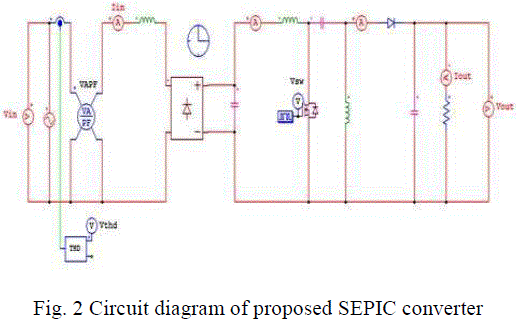 |
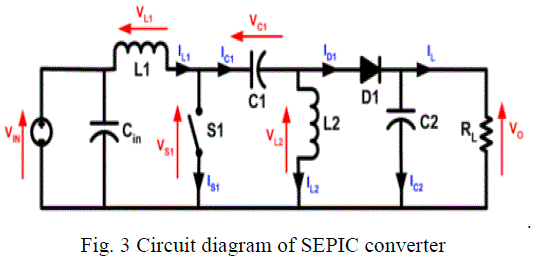 |
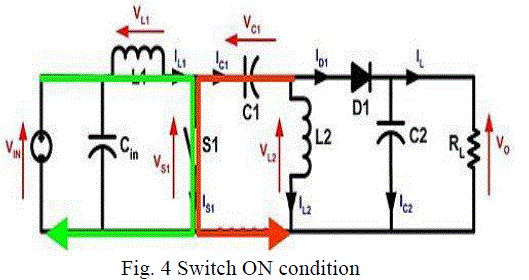 |
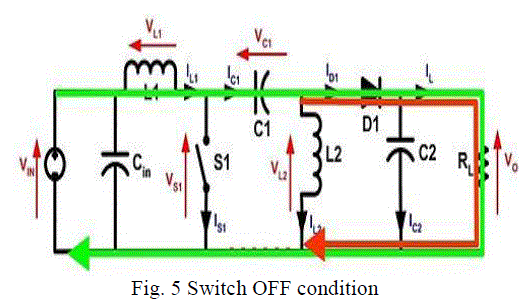 |
Figure 1 |
Figure 2 |
Figure 3 |
Figure 4 |
Figure 5 |
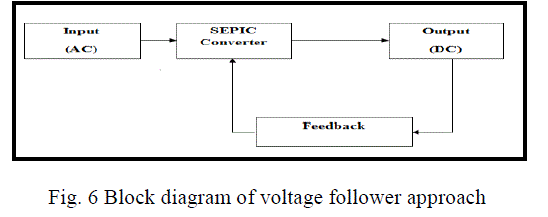 |
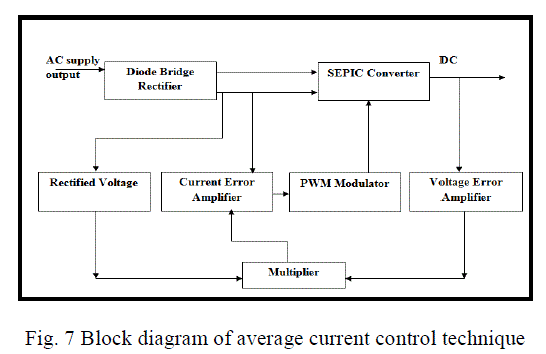 |
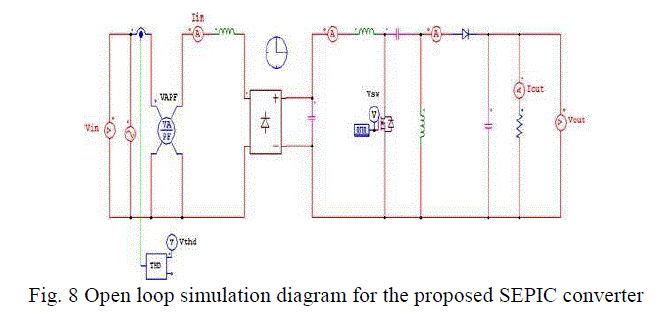 |
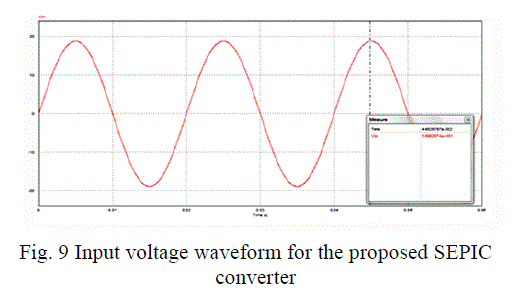 |
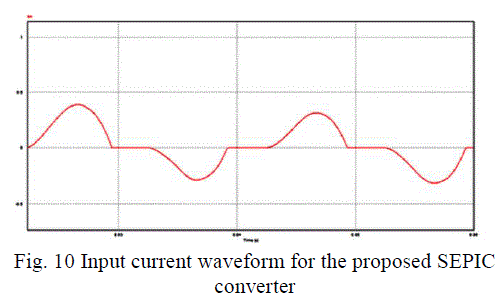 |
Figure 6 |
Figure 7 |
Figure 8 |
Figure 9 |
Figure 10 |
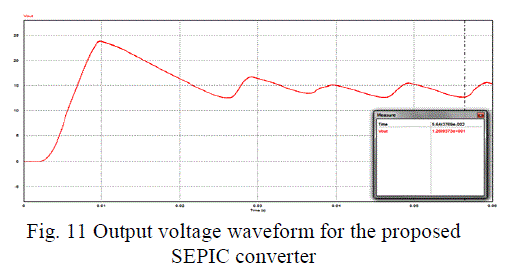 |
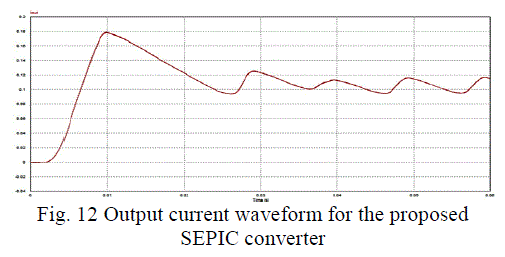 |
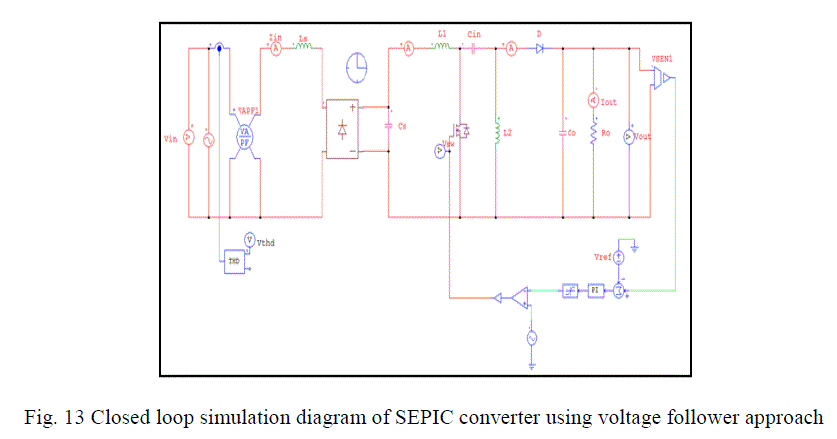 |
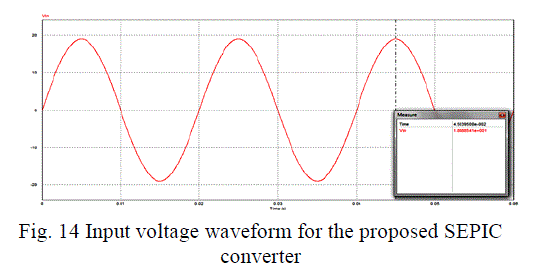 |
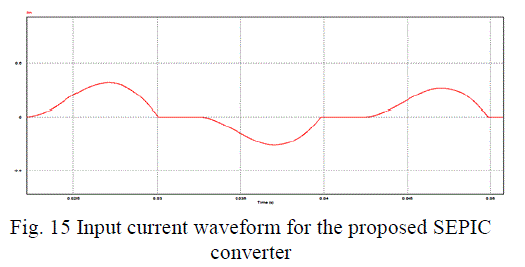 |
Figure 11 |
Figure 12 |
Figure 13 |
Figure 14 |
Figure 15 |
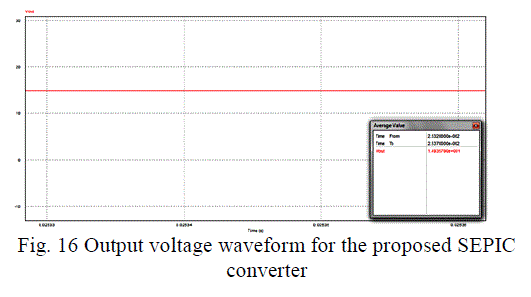 |
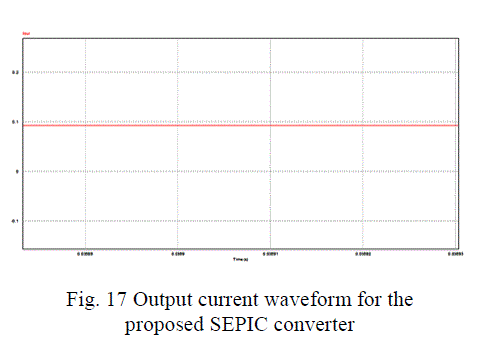 |
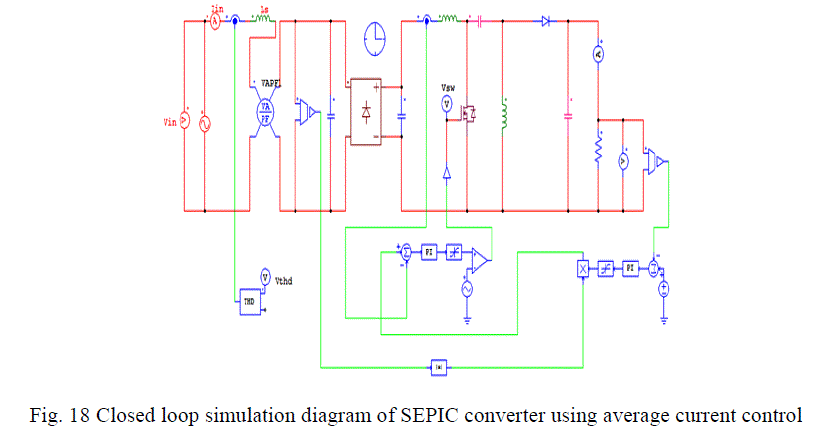 |
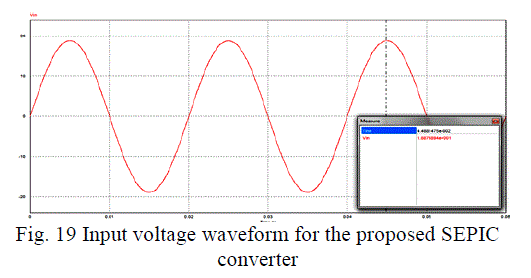 |
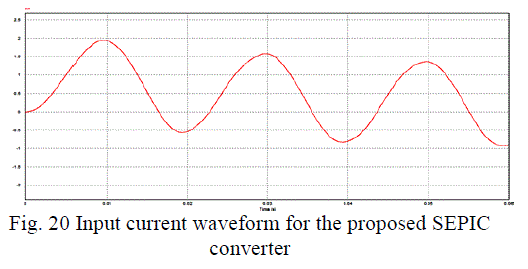 |
Figure 16 |
Figure 17 |
Figure 18 |
Figure 19 |
Figure 20 |
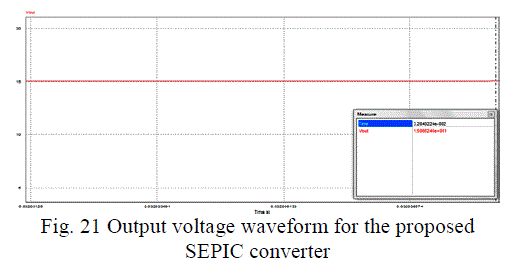 |
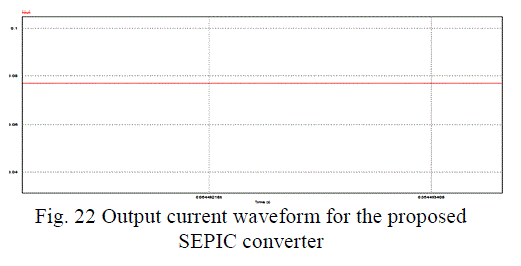 |
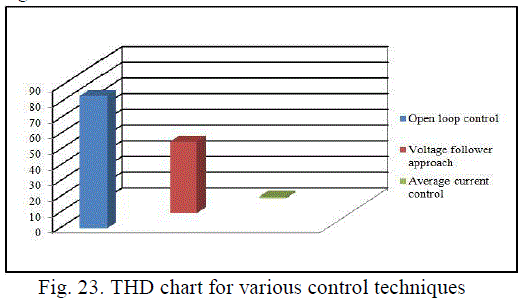 |
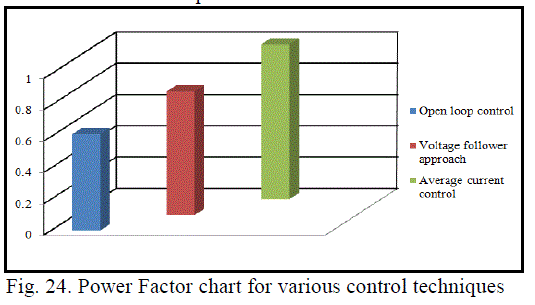 |
Figure 21 |
Figure 22 |
Figure 23 |
Figure 24 |
|
|
References
|
- Biao Zhao, Qiang Song, Wenhua Liu, and Yandong Sun, “Overview of Dual-Active-Bridge Isolated Bidirectional DC–DC Converter for High-Frequency-Link Power-Conversion System”, IEEE Transactions on Power Electronics, Vol.29, No.8, pp. 4091-4106, August 2014.
- R.Ramesh, U.subatra, M.Ananthi, “Single phase AC-DC power factor corrected converter with high frequency isolation using buck converter”, ISSN, Vol.4, No.3, pp. 79-82, March 2014.
- Robert Priewasser, MatteoAgostinelli, ChristophUnterrieder, Stefano Marsiliand Mario Huemer “Modeling, Control, and Implementation of DC–DC Converters for Variable Frequency Operation”, IEEE Transactions on Power Electronics, Vol.29, No.1, pp. 287-301, January 2014.
- Satish. Bandaru, R.Suresh“The Isolated Buck Boost dc to dc Converter With High Efficiency For Higher Input Voltages”, ISSN, Vol.8, No.5, pp. 1-16, December 2013.
- AnamZaman, Gavin Paes, MalaikaD’sa, NipunikaDhawan, Saikrishna V. “Design of a Closed Loop System Using Modified SEPIC” ISSN, Vol.1, No.3, pp. 94-98, April 2013.
- Kavya Shree G V, Eranna, K Cnandra Mohan Reddy, “An Isolated CUK Converter With Multiple Outputs Using PWM Controller” ISSN(Print), Vol.1, No.2, pp. 33-36, 2013.
- ChakibAlaoui, “Spectral Analysis of BUCK and SEPIC Converters”ISSN, Vol.3, No.2, pp. 1705-1711, February 2011
. |