Keywords
|
centre rectangular slot, hexagonal shaped microstrip antenna, left hand and right hand circular polarization, narrow slits, tuning conducting stubs |
INTRODUCTION
|
A standard geometrical shaped patch with a single feed point generally radiate linearly polarized (LP) waves. The CP operation has the distinct advantage over LP of being insensitive to the orientation between the transmitter and receiver, and is therefore widely used in wireless communication systems. In order to radiate CP, it is necessary for two orthogonal patch modes with equal amplitude and in phase quadrature to be excited. A single patch antenna can be made to radiate CP if two orthogonal patch modes are simultaneously excited with equal amplitude and ± 90ïÃâð out of phase with the sign determining the sense of rotation. Single feed CP antennas allows a reduction in the feeding circuit complexity, weight, size and is desirable in situations, where it is difficult to accommodate dual orthogonal feeds with a power divider network [1]-[4]. This can be accomplished by introducing slight perturbation in any regular shaped patches at appropriate locations with respect to the co-axial feed. There are various methods of patch perturbation used to achieve CP viz. embedded slits, slots, matching stubs and defected ground plane. Many single feed patches, using few perturbation methods, have been reported in regular shapes of rectangular [5], circular, square [6] and triangular microstrip antennas [7]. Some gain enhanced antennas have also been studied, but most require increasing antennaâÃâ¬ÃŸs height, such as with parasitic patch [8]. |
The mentioned perturbation methods can be implemented in HMSA to generate CP and is not reported in literature. A hexagonal geometry has area almost equal to that of a circular configuration; the circularly polarized HMSA is expected to have a higher directivity, improved impedance and ARBWs as compared to that of corresponding rectangular or triangular configurations with minor changes in the far field radiation pattern [9], [10]. The previous studies have reported a 4 to 6% [11] impedance BW is achievable with one-layer patch antennas using a thin FR4 dielectric substrate with thickness 1.6 mm and relative permittivity 4.4. Single fed HMSA with various perturbations in the structure can produce circularly polarized waves [12], [13]; despite a drawback of conventional HMSA is a narrowband characteristic and lower antenna gain. To overcome these disadvantages HMSA is used in a stacking configuration. The proposed stacked structure constitutes a driven and a parasitic HMSA with perturbing elements and placed over each other. There are no articles discussed in detail on the stacked HMSA. |
This paper is devoted for the study of various circularly polarized HMSAs with and without stacking for achieving gain and BW enhancement with single coaxial feed. The HMSA are designed and tested experimentally. |
CIRCULARLY POLARIZED HMSA CONFIGURATIONS
|
CP radiations can be achieved by inserting slits, matching stubs along the vertex, and slot at centre of the hexagonal patch. In this section analysis of HMSA with various perturbation techniques for generating CP radiation are designed and studied for antenna parameters like resonant frequency, impedance BW, ARBW, and antenna gain. Figures 1 (a) to (d) depicts the four different configurations of HMSA with single feed to obtain CP using various perturbations. In these configurations the CP radiations can be achieved, when the feed point location and the perturbation length introduced in the patch are optimized, and are finely tuned using simulation tool HFSS –V.11. The feed point location arrangement provides, right hand CP (RHCP) or left hand CP (LHCP). If feed point is located along the diagonal line of the square substrate in the first quadrant with optimized perturbation lengths, then in every configuration of HMSA, LHCP is obtained. The configurations showed in Fig. 1 (a) – (d), yield LHCP waves. The side length, S = 20 mm for all of the HMSA configurations are determined for the MSS band (1990 MHz to 2200 MHz) to resonate at the centre frequency of 2 GHz [14]. The HMSA configurations are etched on a FR4 substrate with dimensions 60 mm x 60 mm, thickness h = 1.59 mm and dielectric constant ïÃÂÃÂ¥r = 4.3, with below side to have a finite ground plane and is fed coaxially by means of a SMA connector. |
A. HMSA with Centre Slot (Antenna 1) |
This is one of the techniques for achieving CP, in which at the centre of HMSA a narrow rectangular slot is embedded in the hexagonal geometry along the x-axis and feeding point is along the diagonal line of the square substrate as shown in Fig. 1(a). Here the electrical length of hexagonal patch remains undisturbed and is equal to that of a simple hexagonal patch, hence its resonating frequency is matching to that of the linearly polarized patch. In this configuration due to the embedded rectangular slot at the centre and by optimizing the feed position along the diagonal line, the HMSA observes an unequal current distribution along the x- and y-axis. Fig. 2 (a) and (b) compares the simulated and measured variations in S11 characteristics with frequency for the HMSA. The embedded rectangular slot has dimensions l x w = 9 mm x 2 mm and is positioned at the centre of the patch. It is due to the centre slot perturbation; the hexagonal patch generates two orthogonal modes of same magnitude but in phase quadrature with each other to resonate at the fundamental centre frequency of 2.16 GHz. The feed position is optimized at the location of (5.2 mm, 5.3 mm) with respect to origin as centre of HMSA. From Fig. 2, the HMSA with centre rectangular slot depicts 10 dB impedance BW of 120 MHz. The 3 dB axial ratio BW for this configuration is measured to be 30 MHz as shown in Fig. 6, and Fig. 7 shows the simulated E-plane and H-plane radiation patterns at 2.15 GHz giving antenna gain of 2.88 dB. |
B. HMSA with two narrow slits at vertex (Antenna 2) |
In this configuration a simple hexagonal patch has two embedded slits of length l and width w, inserted at opposite vertex along x-axis as shown in Fig. 1 (b). The slits create asymmetry in geometry and due to feeding along diagonal line; CP is achieved when proper optimization of the feed point location is performed. Fig. 3 (a) and (b) shows the simulated and measured variation in reflection coefficient with frequency for the case of inserting narrow slits of length l = 9.711 mm and width w = 0.35 mm along x-axis at opposite vertex of HMSA. The ratio of perturbation length to the side length of the HMSA is (l/S) = 0.485. This ratio finds useful at other resonance frequencies for HMSA to obtain CP. With the use of this dimension of the perturbation, a feed location is optimized at a coordinate of (7.5 mm, 7.5 mm) from the centre of the patch along the diagonal line to achieve the two degenerate modes for LHCP and maximum 3 dB ARBW. The CP radiations are observed at the centre resonating frequency of 2.12 GHz, that gives 10 dB impedance BW of 120 MHz, 3 dB ARBW of 31.2 MHz, and gain of 2.74 dB. |
C. HMSA with double stub at vertex (Antenna 3) |
In this case, HMSA geometry constitute of matching conducting stubs along x-axis at both vertex of equal length l, width w and is fed along diagonal line as shown in Fig. 1(c). These stubs create asymmetry in geometry. The stub width is fixed for w = 2 mm where as its length l is varied for impedance matching at the resonating frequency. For the stub length of l = 4.57 mm the CP is achieved at the centre resonating frequency of 2.15 GHz, where the fundamental mode of resonance is degenerated into two orthogonal modes of equal magnitude as shown in Fig. 4 (a) and (b). The feed position is optimized along the diagonal line of the square substrate at the coordinates of (7.6 mm, 7.6 mm) from the centre of HMSA. Here the 10 dB impedance BW of 110 MHz, 3dB AR BW of 20 MHz and antenna gain of 2.646dB are determined. This HMSA also resonates at higher frequency of 3.5 GHz and 4.3 GHz as depicted from the Fig. 4 (a) and (b). |
D. HMSA with single stub at vertex (Antenna 4) |
Likewise the double stub geometry, the single stub geometry is similar as only in this geometry asymmetry is created by the single stub matching at one of the vertex of the hexagonal patch as shown in Fig. 1(d). This type of structure provides additional manufacturing tolerance due to necessity of tuning only one stub at once, than tuning both the stubs simultaneously as discussed in section 2.3. The structure with single stub matching requires a larger stub length than the structure with double stub to achieve CP. Fig. 5(a) and (b) shows the simulated and measured variation in S11 characteristics for the HMSA with single stub matching of length l = 5.57 mm and width w = 2 mm embedded at the vertex along x-axis. The HMSA resonates at the centre frequency of 2.13 GHz giving 10 dB impedance BW of 120 MHz, 3 dB ARBW of 94 MHz, antenna gain of 2.61 dB, when fed along diagonal at the coordinates of (7.15 mm, 5.15 mm) away from the centre of HMSA. |
RESULTS AND DISCUSSION ON VARIOUS CONFIGURATIONS OF HMSA
|
The parametric study for the four types of HMSA is performed in this section with stub matching and insertion of narrow slits at the opposite vertex and at the centre of patch. Table 1 lists the several HMSA parameters with various perturbed geometry for achieving CP. All these four configurations of HMSA are fabricated on a FR4 substrate with ground plane dimensions of 60 mm x 60 mm, having height of 1.53 mm and dielectric constant of ïÃÂÃÂ¥ r = 4.3 and are probe fed along the diagonal line of the square substrate. Fig. 6 shows the comparison of the simulated axial ratio variation with frequency for the four configurations of HMSA. The 3 dB ARBWs are 30 MHz, 31.2 MHz, 20 MHz and 94 MHz for Antenna 1 - 4 respectively. For every HMSA configurations the frequency at minimum axial ratio is matching to that of 10 dB impedance centre frequency. |
This indicates that, the 3 dB ARBW fall within the 10 dB impedance BW and assures CP purity in this range of frequencies. The percent 10 dB impedance BWs and percent 3 dB ARBWs depicted in Table 1 and Table 2 are calculated with respect to the centre frequency of each HMSA configuration. Fig. 7 shows the simulated H- plane and E- plane radiation patterns measured at the centre resonant frequency for all configuration of HMSA. The efficiency of HMSA is 73% for the FR4 substrate which can be improved by using the stacking structure. The Antenna 1 - 4 gives a LHCP radiation pattern providing a total gain of 2.88 dB, 2.74 dB, 2.646 dB and 2.615 dB respectively. The surface current distribution on the patch can be used to identify the sense of polarization of the antenna. If the current vector moves in a clock wise direction, which indicates that the antenna is LHCP. In order to achieve RHCP, the feed has to be shifted by 90ïÃâð and is located in the third quadrant at the same distance from the centre of the patch [12]. Fig. 8 shows the simulated surface current distribution for the HMSA Antenna 1, 2, 3 and 4 at the center resonance frequency of 2.16 GHz, 2.12 GHz, 2.15 GHz and 2.13 GHz respectively. |
STACKED CIRCULARLY POLARIZED HMSA CONFIGURATIONS
|
For CP antennas, stacked patch antennas using parasitic elements are a typical configuration to enhance the BW. By stacking a parasitic patch over the driven patch, BW and gain enhancement of the original patch takes place [15], [16]. The stacked patch antennas have almost same configuration for driven and parasitic patches. Each of the excited and parasitic patches radiates CP waves because the polarization of the parasitic patch depends on that of the driven patch. Single probe fed HMSAs can produce circularly polarized waves by embedding perturbations in the hexagonal structure. A drawback of conventional HMSA is narrowband characteristics of axial ratio. The antenna performance is studied for all configurations of CP HMSA, by stacking it with similar parasitic elements. The side view of the proposed circularly polarized stacked HMSA is shown in Fig. 9. The proposed stacked antenna consists of a hexagonal shaped driven element and a similar hexagonal shaped parasitic element. The driven element is coaxially fed by a 50 ïÃÂÃâ SMA connector. Generally, for CP, the two degenerate mode currents are distributed on the metal surface flowing at the centre of the driven patch. The amplitudes of the two mode currents at each resonant frequency are almost same so that the currents flowing perpendicular to each other ideally would not affect each other. However, the amplitudes for the two current at the same frequency, for example at first degenerate mode, they are not same and the phase difference is not 90ïÃâð. Hence, the driven patch itself does not radiate circularly polarized waves. The equivalent network of the stacked configuration composes of two resonators and one reactance. The reactance is divided by two orthogonal currents, which represent resonant phenomenon to each orthogonal direction. The two resonators represent the resonance caused by the driven and parasitic element, and the reactance represents the coupling between the elements [17]. In this section a study is performed by the use of stacked HMSA to determine the antenna parameters after stacking it with a similar parasitic patch. In this analysis, all configurations of HMSA as discussed in section 2 are stacked with the same type of driven patch of similar side length dimensions (S = 20 mm) and their results are compared. The investigation of these parameters is detailed in Table 2 for comparison with the parameters of Table 1. |
From Table 2 for Antenna1 the HMSA with centre slot when stacked with the same type of HMSA; this antenna observes the 10 dB impedance BW of 8.7 %, the 3 dB ARBW of 3 % and gain of 2.986 dB. The stacked HMSA antenna observes an increment in these parameters by 3.15 %, 1.62 % and 0.106 dB respectively; in comparison to HMSA before stacking with same parasitic element. In the Antenna2 for HMSA embedded with narrow slits at opposite vertex when stacked with the same type of HMSA; the stacked antenna observes the 10 dB impedance BW of 7.59 %, the 3 dB ARBW of 2.82 % and gain of 2.89 dB. After stacking with the same type of parasitic element, the single layer HMSA observes an increment of 1.93 %, 1.35 % and 0.15 dB respectively. In the Antenna 3 the 10 dB impedance BW before stacking parasitic element was 5.1 % and BW after stacking with same parasitic element is 7.66 % so a 2.56 % BW enhancement takes place due to stacking. The 3 dB ARBW and gain of Antenna 3 are also getting improved by 3.46 % and 0.244 dB respectively. Similar characteristics are found for Antenna 4 for the stacked HMSA with single stub; the 10 dB impedance BW enhancement of 2.69 %, 3 dB ARBW improvement of 0.41 % and gain increases by 0.146 dB in comparison with its corresponding unstacked HMSA. |
CONCLUSION
|
Four structures for generation of CP in a HMSA of side length 20 mm is studied so that the hexagonal shaped patch resonates at centre frequency of 2 GHz in the MSS band. In these structures the antenna is probe fed along its diagonal and the perturbations either are in the form of a rectangular slot at centre, with two narrow slits at opposite vertices, double conducting stub at opposite vertices and with a single conducting stub at one of the vertex. Four such hexagonal antenna configurations are designed, fabricated and measured to find that the antenna radiates LHCP waves. To enhance the antenna parameters in their single layer structure, stacking the driven antenna by the similar parasitic patch element finds useful. On an average the overall improvement in the 10 dB impedance BW, 3 dB ARBW and gain for the HMSA configurations due to stacking with the similar type of parasitic elements is found to be 2.58%, 1.71 % and 0.1615 dB respectively. Theoretical results for the reflection coefficient have been validated with experiments. |
ACKNOWLEDGMENT
|
The authors are thankful to the Vice- Chancellor of DIAT and Shivaji University and to the Principal, Sinhgad Academy of Engineering, for providing the lab facilities and all the necessary support. |
Tables at a glance
|
 |
 |
Table 1 |
Table 2 |
|
|
Figures at a glance
|
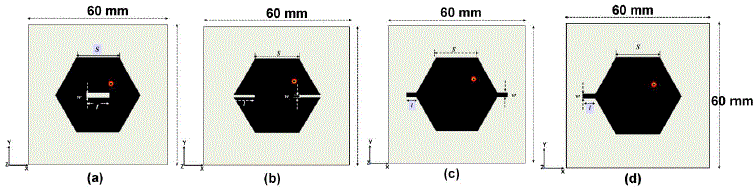 |
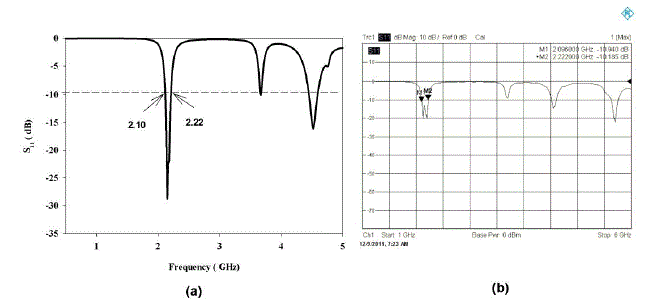 |
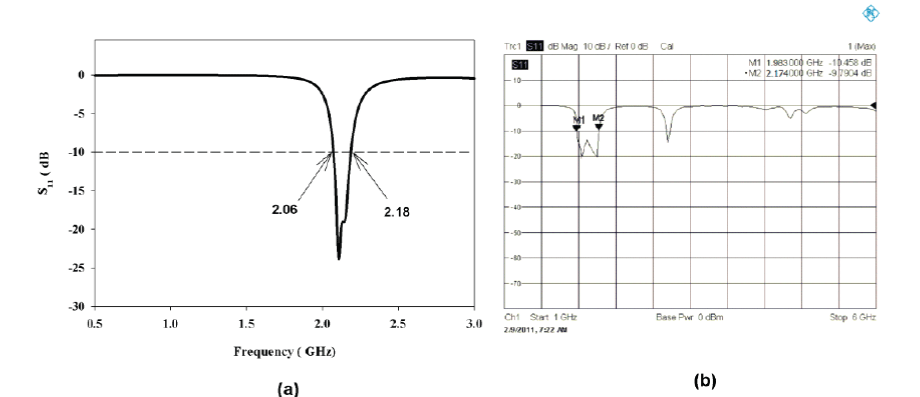 |
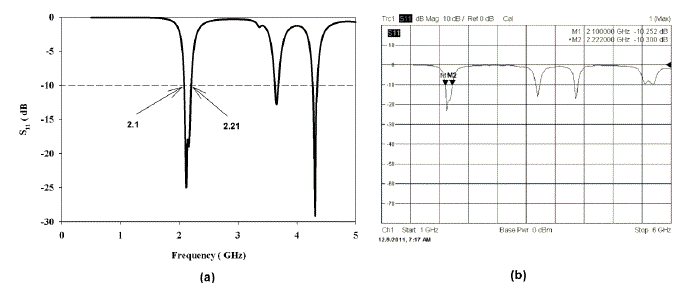 |
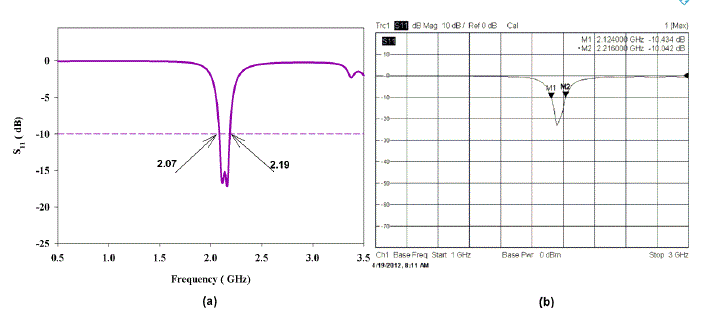 |
Figure 1 |
Figure 2 |
Figure 3 |
Figure 4 |
Figure 5 |
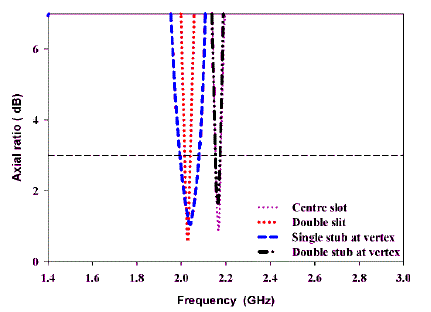 |
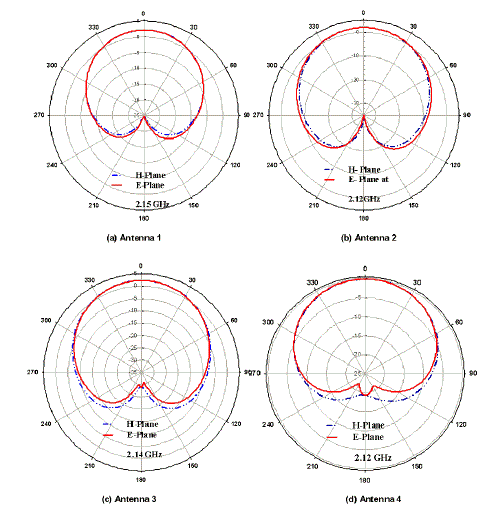 |
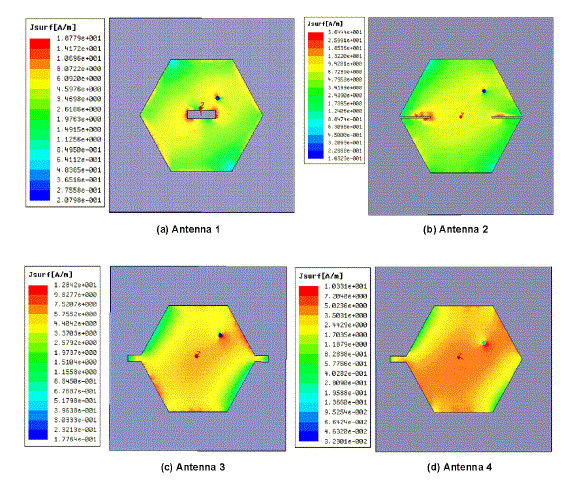 |
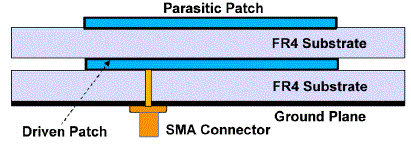 |
Figure 6 |
Figure 7 |
Figure 8 |
Figure 9 |
|
|
References
|
- Kumar G. and K.P. Ray, “Broadband Microstrip Antennas,” Artech House Publishers, London, pp. 205–247, 2003.
- Wong, K. L. “Compact and Broadband Microstrip Antennas,” John Wiley & Sons, Inc., pp. 45–156, 2002.
- Volakis, J. L. “Antenna Engineering Handbook,” McGraw-Hill publications, pp. 73-97, 2007.
- Sharma, P. C. and K. C. Gupta, „„Analysis and Optimized Design of Single Feed Circularly Polarized Microstrip Antennas,âÃâ¬ÃŸÃ¢Ãâ¬ÃŸ IEEE Trans.Antennas Propagation, Vol. 31, No. 6, pp, 949–955, 1983.
- Deshpande, M. D. and N. K. Das, „„Rectangular Microstrip Antenna for Circular Polarization,âÃâ¬ÃŸÃ¢Ãâ¬ÃŸ IEEE Trans. Antennas Propagation, Vol. 34, No. 6, pp. 744–746, 1986.
- Wong, K. L. and J. Y. Wu, „„Single Feed Small Circular Polarized Square Microstrip Antenna,âÃâ¬ÃŸÃ¢Ãâ¬ÃŸ Electronics Letters, Vol. 33, No. 22, pp. 1833–1834, 1997.
- Tang, C. L., J. H. Lu, and K. L. Wong, “Circularly polarized equilateral triangular microstrip antenna with truncated tip,” Electron. Lett., vol. 34, pp. 1277–1278, 1998.
- Luk, K. M. K. F. Tong, and T. M. Au, “Offset dual-patch microstrip antenna,” Electron. Lett.,vol. 29, pp. 1635 - 1636, 1993.
- Martin, N. and Griffin, D., “A new approach to microstrip antenna BW determination and its application to a novel hexagonally shaped patch,” Antennas and Propagation Society International Symposium, 175-178, May 1982.
- Arvind, K.S. and J.R. Wolfgang, “Spectral domain analysis of a hexagonal microstrip resonator”, IEEE Trans Microwave Theory Techniques,vol.30, pp. 825–828, 1982.
- Huang, C. Y., J. Y. Wu, and K. L. Wong, “ High-gain compact circularly polarized microstrip antenna,” Electron Lett., vol. 34, pp. 712– 713,1998.
- Ray, K. P., D. M. Suple and N. Kant, “Perturbed Hexagonal Microstrip Antenna for Circular Polarization,” IEEE, 978-1-4244-4819-7/09, 2009.
- Ray, K. P., D. M. Suple and N. Kant, “Suspended Hexagonal Microstrip Antennas for Circular Polarization,” International Journal ofMicrowave and Optical Technology, vol.5, No. 3, pp. 119-123, May 2010.
- Ray, K. P., M. D. Pandey and S. Krishnan, “Determination of Resonance Frequency of Hexagonal and half Hexagonal Microstrip Antennas,”Microwave And Optical Technology Letters, vol. 49, No. 11, pp. 2876-2879, November 2007.
- Choi, W. C., Pyo and J. Choi, “Broadband circularly polarized corner truncated square patch array antenna,” IEEE Antennas and PropagationInt . Symp.,San Antonio, pp.220-223, 2003.
- Noro, T. and Y. Kazama, “A Novel wideband circular polarization microstrip antenna – combination of different shaped antenna element,” IEEE Antennas and Propagation. Int. Symp., Washington, DC, pp. 460-467, 2005.
- NoroTakanori, Yasuhiro Kazama, Masaharu Takahashi and Koichi Ito, “A Study on the Mechanism of Wideband Characteristics for Single-Fed Stacked Circularly Polarization Patch Antenna,” IEEE Conference proceedings of 1-4244-0878-4/07, pp. 733-736, 2007
|