As the phenomenon of electricity of alternating in nature is known by us, we can stated that the AC energy cannot be stored for the long period of time, except that it can be taking as short duration storing quantity. But it has the property that it can consume and used at the same time. So that for the power plant it is necessary to produce continuously and constant power to avoid fluctuations in it.Power system protection or control is based on the same principle that in casing of a disproportion, the frequency of the AC network changes. During the working period of grid, the frequency should be kept constant to avoid the unbalancing condition. But the variation can be tolerated within certain limits.In this work we study the causes of frequency fluctuations, and explore the possibility of assembling information about the state of the system and hence the controlled performance increases by these fluctuations being measured. We reach the conclusion that due to random demand of system frequency fluctuations occurs, but their nature is also influenced by the state of the generators that are participating in power control.
Keywords |
Frequency fluctuation, balancing of frequency, control techniques. |
INTRODUCTION |
Energy storage systems are mainly used to store the energy to use it later for power requirements indifferent systems.
Therefore, they can play a useful role in power system when electric power is most needed and most valuable. For the
next generation integrated power system the next challenges that has to meet are many but one of them is design of
such power source that meet a growing ship of power requirement and that should be in very efficient manner possible.
The power sources included by large synchronous generators, hundreds of mega joules of dedicated energy storage for
pulsed loads, and over 100 MJ of total energy in battery operated uninterruptible power supplies (UPS) distributed
throughout the ship’s load centres (NAVSEA, 2007). |
The Naval Sea Systems Command (NAVSEA,2007) has identified the functions of an integrated energy storage
system, as supporting some loads during a loss of power generation, mitigating the system effect of a large load step,
and enabling the provision of a large pulse of power to a pulsed load without applying that pulse to the overall power
system. The second function addresses sudden changes in generator output power demand, such as during the charging
of pulse load energy storage systems for electromagnetic weapons or aircraft launchers, propulsion motor power
reversal during ship crash astern maneuvers and thetripping offline of generators due to AC system faults [1]. The
power systems majorly affect by the sudden change in electrical loading on the synchronous generators can cause their
frequency of the output power frequency to fluctuate widely as the prime mover adjust to meet the demand. |
The maximum that can be tolerated, rate per second for typical gas turbines is estimated by NAVSEA (2007) to be
20%. Fig. 1 shows the virtual load acceptance response of a 45 MVA synchronous generator (inertia constant of 4.0
MWs/MVA) with an aero derived, gas turbine prime mover. The acceptable generator frequency fluctuation are
measured that is ±4% limits for transitory frequency tolerance specified in MILSTD1399 (NAVSEA, 1987) and
mirrored in IEEESTD45 (IEEE, 2002). They offer a prospective performance benchmark for energy storage integration
schemes that deal with load step disturbances. |
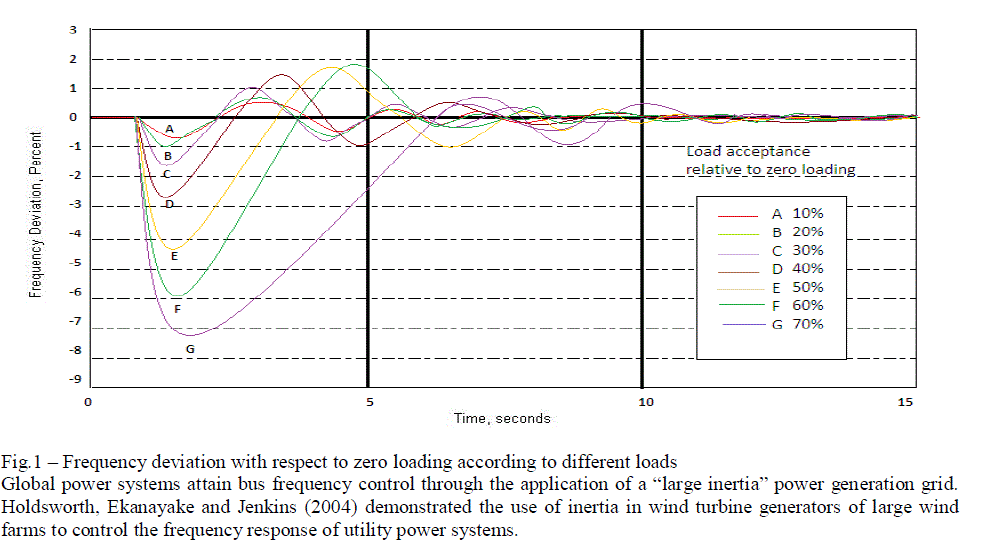 |
LITERATURE SURVEY |
Today frequency and voltage control mostly occurs at the AC transmission level. In distributed systems with some
additional circuits these control functions take place both at the transmission and distribution levels [1]. As the demand
increases the consumption of power with respect to desired load fluctuates so that the burden on prime mover of the
generator oscillates. Sometimes the burden causes the frequency deviation with respect to its zero loading line. As
much as the demand or burden increases the fluctuation in frequency increases, hence greater the load will produce
greater fluctuation in frequency. A recently published article [2] indicated that the German grid might be stressed (e.g.,
large frequency and voltage deviations) even at light-load conditions if the 26 nuclear plants are permanently
disconnected. Distributed central stations (nuclear, coal and natural gas) ensure that the power-flow control is relatively
stable because energy must not be transmitted over long (e.g., 800km) lines to reach the consumer. These grids are
connected to maximize stability, efficiency and reliability while minimizing investment costs. In Germany, the fact that
wind-power (WP) plants are predominantly located in the north (mainly offshore in the North Sea) and photovoltaic
(PV) plants are mainly located in the south means that the electric energy must be transmitted over a long distance
(about 800km) resulting in unacceptably large frequency and voltage perturbations [2], [3] which lead to stability
problems due to large transmission-line impedances and switching actions. In the year 2012 the northern grid of India
was blackout and the reason that founded in investigation of problem is because of frequency variation (The new
frequency we got at that time was 49.76Hz, the maximum value of which is 50.60 and the minimum value we have got
that is 48.88Hz). |
Variation Control Mechanism: |
Frequency balancing and control operation can be performed in time domain using different resources, represented in
figure 2. |
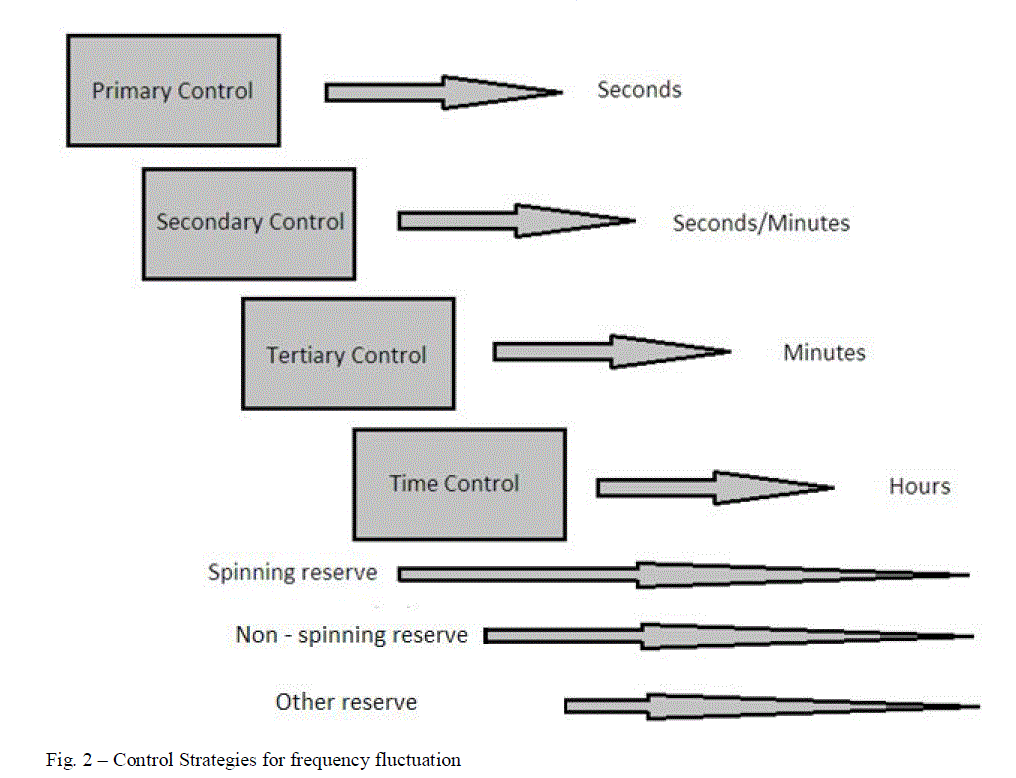 |
Primary Control |
Primary Control is more generally known as Frequency Response. An external control loopcalled governor control,that
is contained by each turbine prime mover of a conventional generator is typically equipped with it. Governor control
system controls the rotation speed of the shaft by altering thesupply to the turbine and thus control frequency. This type
of control is usually called primaryfrequency control. [6]Frequency Response takes place within the first few seconds
following a change in system frequency (disturbance) to stabilize the Interconnection. Frequency Response is provided
by the following: |
1. Governor Action. Governors on generators are similar to speed control on your car. A change in speed is sensed by
them and adjusts the energy input into the generators’ prime mover. |
2. Load. In an Interconnection change in the speed of the motor is directly proportional to frequency. As frequency
drops, motors will spin slower and draw less power. The reduction of system load (rapidly) may also be effected by
automatic operation of under-frequency relays which break off pre-defined loads within fractions of seconds or within
seconds of frequency reaching a predetermined value. Such reduction of load may be represented as interruptible load.
As a safety net, percentages of firm load may be dropped by under-frequency load detaching programs to ensure
stabilization of the systems under severe disturbance scenarios. |
It is important to be remembered that Primary Control will not return frequency to normal, but only stabilize it. Other
control components are used to restore frequency to normal. |
Secondary Control |
The balancing services deployed in the secondary control is typically includes the “minutes” time frame. On the other
hand some resources, such as hydroelectric generation, be able to respond faster in many cases. Secondary Control also
includes preliminary reserve deployment for instability. Terms most frequently associated with this are “Load-
Frequency Control” or “Automatic Generation Control”. In short, Secondary Control maintains the minute-to-minute or
second-to-second balance all over the day and is used to restore frequency to its scheduled value, usually 50 Hz,
following a disturbance. Secondary Control is provided by both Spinning and Non-Spinning Reserves. The most
common resources of exercising secondary control is through Automatic Generation Control (AGC). AGC operates in
combination with Supervisory Control and Data Acquisition (SCADA) systems. Gathering of information is done by
SCADA in an electric system, in particular generator outputs, system frequency and actual interchange between the
system and neighboring systems. Using system frequency and net actual interchange, plus knowledge of net scheduled
interchange, it is feasible to determine the system’s energy balance with its interconnection in near-real-time. AGC
describes a Balancing Area’s Area Control Error (ACE, further described below) from interchange and frequency data.
ACE tells whether a system is in synchronism or desires to make adjustments to generation. |
The level of success of AGC in complying with balancing and frequency control is manifested in a Balancing Area’s
control performance compliance statistics, which are described in greater detail later in this document. |
Tertiary Control |
In the tertiary frequency control, manual and automatic changes take place in the dispatching and commitment of
generating units. This control is used to reestablish the primary and secondary frequency control reserves, to manage
overcrowdings in the transmission network, and to bring the frequency and the interchanges back to their target value
when the secondary control is incapable to achieve this last task. [10]The action taken for tertiary Control encompasses
to get resources in place to handle current and future contingencies. Reserve deployment and Reserve re-establishment
following a disturbance are common types of Tertiary Control. |
Time Control |
The balancing control schemes for Frequency are not perfect. There will always be asymmetrical errors in tie-line
meters whether due to transducer inaccuracy, problems with SCADA hardware or software, or communications errors.
Due to these errors, generation deviation and normal load fluctuation, net ACE in an Interconnection cannot be
maintained at zero. This means that frequency cannot always be maintained at exactly 50Hz, and that normal (or
average) frequency over time usually is not exactly 50 Hz. |
In electrical power system each interconnection has a Time Control process to maintain the long-term normal (or
average) frequency at 50 Hz. While there are some differences in process, each interconnection designates a
consistency manager as a “Time Monitor” to provide Time Control. The Time Monitor comparison takes place with a
clock driven off Interconnection frequency against “official time” provided by the National Institute of Standards and
Technology (NIST). If average frequency drifts, it creates a Time Error between these two clocks.
For example, if frequency has been running 2 mHz high (50.002Hz), a clock using interconnection frequency as a
reference will gain 1.44 seconds in a 10 hour interval (i.e., 50.002 Hz-50.000 Hz)/50 Hz * 10 hrs * 3600 s/hr = 1.44 s).
If the Time Error accumulates to a pre-fixed value (for this example, +10 seconds in the Eastern Interconnection), the
Time Monitor will send notices for all Balancing Authorities in the interconnection to compensate their scheduled
frequency by -0.02Hz (Scheduled Frequency = 49.98Hz). This offset, known as Time Error Correction, will be
maintained until Time Error has decreased below the termination threshold. |
RESULT& DISCUSSION |
This paper is basically focused on the generation and termination of fluctuation or disturbance in power system
frequency. Which can be shifted due to some reasons like loading, unloading frequency mismatching etc. and it cannot
be tolerated by any system for a long duration. To maintain synchronism it is very important that the frequency should
be maintained during running condition of any healthy system. By analysing data in graph of figure 1 will give
information that with respect to zero loading the system frequency can how much fluctuate and the time collapse to
regain its synchronism. The table including information for figure 1 is as follows: |
 |
 |
The power system is based on power generation and its consumption. In one system there always should be a common
frequency but frequency changes and its fluctuation can be reduced using different methods. We here discussed about
some methods we use recently but it needs to increases that protection level so that the sharpness and accuracy of the
protective elements are the two major things what we have to improve in future. |
FUTURE SCOPE |
Our work will focus on stabilization of system from unwanted disturbance produced by over loading, load shading and
sudden removal of load etc. The frequency directly proportional to the load applied to the system. The prime mover of
generator gets disturbed when the system unbalanced from loading. The present arrangement for maintaining
synchronism in the system is not so accurate for the instant responding to faults. This paper is based on the problems
associated with the system when the frequency fluctuates from its zero loading line. In future the systems will be based
on the AGC and AEC controlling schemes so that the protection techniques can work in instant and the faulty
conditions can be avoided as much as possible. |
CONCLUSION |
Control strategies for minimizing Integrated Power System MV bus frequency variations all through generator load
steps have been presented. They consist of using frequency variation to manage power demand of the propulsion
motors, regenerative power output from the propulsion motors, and regenerative power output from a dedicated
capacitor energy storage device. |
The compensation of frequency variation is possible only when the load is stable so that the synchronism in any power
system can be maintained. For the industrial areas the demand of the power always varies with the load, so that these
variations primarily maintain by neighbouring system or the prime mover setting. The above mentioned techniques can
be used to balance the fluctuation but are not so much efficient that they can withhold the whole system disturbance. |
References |
- E.F. Fuchs and M.A.S. Masoum, Power Quality in Power Systems and Electrical Machines, Elsevier/Academic Press, Feb. 2008, 638 pages.ISBN 978-0-12-369536-9.
- http://www.n-tv.de/politik/Stromnetz-leidet-unter-Stress-article3485686.html
- E.F. Fuchs and M.A.S. Masoum, Power Conversion of Renewable Energy Systems, Springer, USA, April 2011, 692 pages. ISBN: 978-1- 4419-7978-0..
- Janice Lin, Giovanni Damato and Polly Hand, âÃâ¬ÃÅEnergy StorageâÃâ¬Ãâa Cheaper, Faster, &CleanerAlternative to Conventional Frequency Regulation,âÃâ¬Ã Prepared for the California Energy StorageAlliance, February 16, 2011.Available at: http://www.iceenergy.com/stuff/contentmgr/files/1/76d44bfc1077e7fad6425102e55c0491/download/cesa_energy_st orage_for_frequency_regulation.pdf
- Sandip Sharma, Shun-Hsien Huang, and NDR Sarma, âÃâ¬ÃÅSystem Inertial Frequency ResponseEstimation and Impact of Renewable Resources in ERCOT Interconnection,âÃâ¬Ã IEEE Power andEnergy Society General Meeting, 24-29 July 2011.
- Christopher L. DeMarco, Chaitanya A. Baone, Yehui Han, and Bernie Lesieutre, âÃâ¬ÃÅPrimary andSecondary Control for HighPenetration Renewables,âÃâ¬Ã PSERC Publication, March 2012.
- Hassan Bevrani, Robust Power System Frequency Control, Springer, 2009.
- KEMA Inc., âÃâ¬ÃÅResearch Evaluation of Wind Generation, Solar Generation, and Storage Impact on the California Grid,âÃâ¬Ã Prepared for the California Energy Commission, Public Interest Energy Research Program, June 2010. Available at: http://www.energy.ca.gov/2010publications/CEC-500-2010-010/CEC-500-2010-010.PDF
- FERC Order No. 755, âÃâ¬ÃÅFrequency Regulation Compensation in the Organized Wholesale Power Markets,âÃâ¬Ã Issued October 20, 2011. Available at: http://www.ferc.gov/whats-new/comm-meet/2011/102011/E-28.pdf
- Yann G. Rebours, Daniel S. Kirschen, Marc Trotignon, and S̮̩bastienRossignol âÃâ¬ÃÅA Survey offrequency and voltage control ancillary services part I: Technical features,âÃâ¬Ã IEEE Transactions onPower Systems, Vol. 22 , Issue: 1 , February 2007.
|