Keywords
|
Flexible AC Transmission System (FACTS), Static Var Compensator (SVC), PSAT, Voltage Stability, Voltage Stability Index (VSI). |
INTRODUCTION
|
Most of the large power system blackouts, which occurred worldwide over the last twenty years, which are caused by heavily stressed system with large amount of real and reactive power demand and low voltage condition. When the voltages at power system buses are low, the losses will also to be increased. This study is devoted to develop a technique for improving the voltage and eliminate voltage instability in a power system. Application of Flexible AC Transmission System (FACTS) devices are currently pursued very intensively to achieve better control over the transmission lines for manipulating power flows. They can provide direct and flexible control of power transfer and are very helpful in the operation of power network. The power system performance and the power system stability can be enhanced by using FACTS device [1]. |
FACTS devices, which are power electronic based devices can change parameters like impedance, voltage and phase angle. They also helps to reduce flows in heavily loaded lines, resulting in an increased load ability, low system loss, improved stability of the network, reduced cost of production and fulfilled contractual requirement by controlling the power flows in the network. They provide control facilities, both in steady state power flow control and dynamic stability control [2]. The possibility of controlling Power flow in an electrical power system without generation rescheduling or topological changes can improve the performance considerably. Static Var Compensator (SVC) is one of the most effective device for enhancing the power stability and power transfer capability of transmission network, for this the SVC should be properly installed in the system with appropriate parameter setting. The some factors considering for optimal installation and the optimal parameter of SVC , which are the stability margin improvement, power loss reduction, power blackout prevention and the power transmission capacity enhancement [3]. |
This paper presents the method of the optimal location of SVC for enhancing the voltage stability of the power system using VSI. The SVC has been tested on the 9-Bus test System and the solution for optimal location of this device will obtain and discuss in this section. |
Shravana Musunuri, Gholamreza Dehnavi [1], compares STATCOM, SVC, SSSC and TCSC devices for steady state voltage stability improvement. The choice of the location ,sizing and cost comparison of these FACTS devices with traditional reactive power/voltage stability devices are presented. Bhavin. M. Patel [2], explained the enhancement of steady state voltage stability using SVC and TCSC. The results presented in this paper clearly show how SVC and TCSC can be used to increase system stability in practical power systems with the use of simulink model. Rambabu, Y.P.Obulesu, Saibabu [3], aimed for improvement of voltage profile and reduce power system losses by using multi type FACTS devices. Their individual contribution towards the improvement of voltage profile and reduction of power losses has been tested on a 5-bus system. P. Ajay D. Vimal Raj, M. Sudhakaran [4], proposed optimum load shedding in power system strategies with voltage stability indicators. In this paper a fast method for determining the location and quantity of the load to be shed in order to avoid risk of voltage instability is presented. P. Kessel and H. Glavitsch [5], estimate the voltage stability of a power system. This method is based on the indicators of risk of voltage instability. This paper proposes a method to compute load curtailment evaluation, using optimal power flow computation, incorporating the steady state voltage stability margin constraints. N.Boonpirom and K.Paitoon Wattanakij [6], presented the static voltage stability enhancement using FACTS devices. With the insertion of SVC, enhancement in voltage at various buses, reduction in system power loss, improvement in power factor and power flow are the objectives of the study. Mehrdad Ahmad, Mostafa Alinezhad, [7] Compares SVC and STATCOM for the static voltage stability margin enhancement, The choice of the location and sizing of these devices is also presented and an analysis is made on the IEEE 14 bus system using Power System Analysis Toolkit (PSAT) software. |
MATHEMATICAL MODEL OF SVC
|
In this paper steady state model of FACTS devices are developed for power flow studies. SVC modelled is incorporated into the bus as shunt element of transmission line. Mathematical model for SVC is implemented by MATLAB programming language. |
Static VAR compensator (SVC)
|
The SVC uses conventional thyristor to achieve fast control of shunt-connected capacitors and reactors. The configuration of the SVC is shown in Fig.1, which basically consists of a fixed capacitor (C) and a thyristor controlled reactor (L). The firing angle control of the thyristor banks determines the equivalent shunt admittance presented to the power system. |
A shunt connected static VAR generator or absorber whose output is adjusted to exchange capacitive or inductive current so as to maintain or control bus voltage of the electrical power system. Variable shunt susceptance model of SVC [2] is shown in Fig.1 |
As far as steady state analysis is concerned, both configurations can modelled along similar lines, The SVC structure shown in Fig. 1 is used to derive a SVC model that considers the Thyristor Controlled Reactor (TCR) firing angle as state variable. This is a new and more advanced SVC representation than those currently available. The SVC is treated as a generator behind an inductive reactance when the SVC is operating within the limits. The reactance represents the SVC voltage regulation characteristic. The reason for including the SVC voltage current slope in power flow studies is compelling. The slope can be represented by connecting the SVC models to an auxiliary bus coupled to the high voltage bus by an inductive reactance consisting of the transformer reactance and the SVC slope, in per unit (p.u) on the SVC base. A simpler representation assumes that the SVC slope, accounting for voltage regulation is zero. This assumption may be acceptable as long as the SVC is operating within the limits, but may lead to gross errors if the SVC is operating close to its reactive limits [3]. |
The current drawn by the SVC is,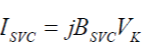
|
The reactive power drawn by SVC, which is also the reactive power injected at bus k is, |
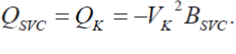 |
Where, VK – voltage at bus k |
ïÃâ¬Ã SVC B Susceptance |
ïÃâ¬Ã SVC Q Reactive power drawn by SVC |
OPTIMAL LOCATION OF FACTS DEVICES
|
To improve the voltage profile and voltage stability of a power system an alternative solution is to locate an appropriate Flexible AC transmission system (FACTS) device [2]. FACTS devices are the solid state converters having capability of improving power transmission capacity, improving voltage profile, enhancing power system stability, minimizing transmission losses etc. In order to optimize and to obtain the maximum benefits from their use, the main issues to be considered are the type of FACTS devices, the settings of FACTS devices and optimal location of FACTS devices. |
The optimal location of SVC has been selected on the basis of voltage stability index (VSI) for improvement of voltage stability of power system [6, 7]. Voltage stability index can be used for determining the weakest line in a power system. This voltage stability index considers both active and reactive powers to evaluate voltage stability, which provides information about the stability condition of the lines and also determines the weakest line in the system. |
VOLTAGE STABILITY INDEX
|
Voltage stability is becoming an increasing source of concern in secure operating of present-day power systems. The problem of voltage instability is mainly considered as the inability of the network to meet the load demand imposed in terms of inadequate reactive power support or active power transmission capability or both. It is mainly concerned with the analysis and the enhancement of steady state voltage stability based on L-index. This L-Index [3] determines how any system is close to its instability limit. |
Consider, a simple system consisting of sending and receiving end buses as shown, |
Where: VS, VR = sending and receiving voltages at system buses |
δ1, δ2 = sending and receiving voltages angle at system bus 1 and 2 |
P, Q = real and reactive power at buses |
R+ JX = line impedance between bus 1 and 2. |
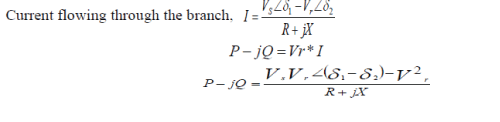 |
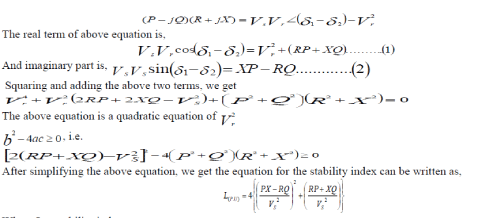 |
Where L = stability index |
For stable system, L< 1. |
It can be seen that when a load bus approaches a steady state voltage collapse situation, the L-index approaches the numerical value 1.0. Hence for an overall system voltage stability condition, the index evaluated at any of the buses must be less than unity. Thus the index value L gives an indication of how far the system is from voltage collapse [6]. |
RESULT AND DISCUSSION
|
For the validation of the proposed FACT’s devices, SVC have been tested on the WSCC 9-Bus test System. A MATLAB code for both techniques was developed for simulation purpose. |
An WSCC 9 bus test system and this test system including 9 buses ,3 generators ,6 lines ,3 transformers and 3 loads is simulated using PSAT .and used to try models and control strategies is presented. The generators are modelled as standard PV buses with both P and Q limits; loads are represented as constant PQ loads. The P and Q load powers are not voltage dependent and are assumed to change as follows |
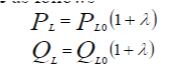 |
Where PLo and QLo are the active and reactive base loads. Whereas PL and QL are active and reactive loads at bus L for the current operating point as defined by λ. |
Location of SVC
|
Voltage stability indices are calculated for the WSCC 9 bus system without SVC as shown in table 2.By considering the Voltage stability index (L-Index) value, it is observed that line 7-5 is more sensitive towards system security. Therefore it is more suitable location for SVC to improve power system security/stability. After placement of SVC voltage stability is improved, the analyses of voltage magnitudes for WSCC 9 bus test system without and with SVC as shown in table 3. |
SIMULATION RESULTS
|
Base case
|
The continuation power flow analysis using PSAT [12] is run for the test system and the voltage profile without SVC is obtained. The voltage profile and PV curve for the bus 5, bus 6and bus7. It can be seen from the figure 4 (a) that the maximum loading parameter ïÃÂì ïÃâ¬Ã½ 1.8708 p.u for the base case. |
With SVC
|
It is seen from Fig. 4(b) that the voltage collapse occurs at the maximum loading of ïÃÂì ïÃâ¬Ã½1.8708 and line 7-5 is the best location for shunt compensation. As described in the earlier section, the SVC was placed in the weakest bus lines one at a time and it was found that for this system bus line 7-5 shunt compensation has better results. Fig.5 (a) gives the voltage profile for the system with SVC. It is seen that the maximum loading factor ïÃÂì ïÃâ¬Ã½1.9815has increases when compared to the base case. |
CONCLUSION
|
In this paper, a new method for optimal placement and parameters settings of SVC has been proposed for improving voltage profile in a power system. The proposed approach has been implemented on WSCC 9-bus system. The criterion for selection of optimal placement of SVC was to maintain the voltage profile, minimize the voltage deviations and to reduce the power losses using VSI. Simulations performed on the test system shows that the optimally placed SVC maintains the voltage profile, improve the stability and effectively increases the efficiency of power system. |
Tables at a glance
|
|
Figures at a glance
|
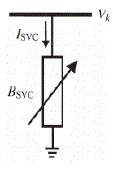 |
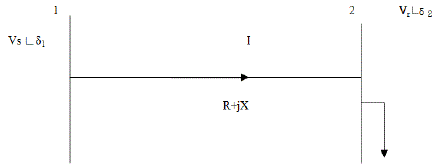 |
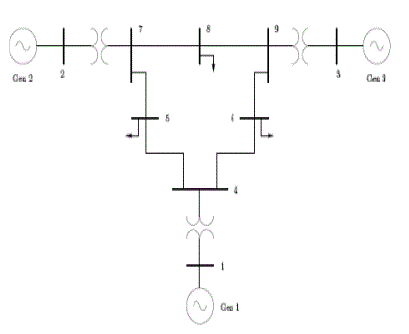 |
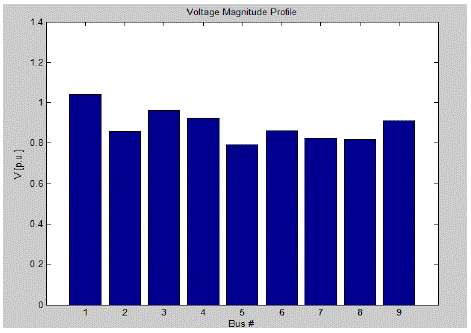 |
Figure 1 |
Figure 2 |
Figure 3 |
Figure 4 |
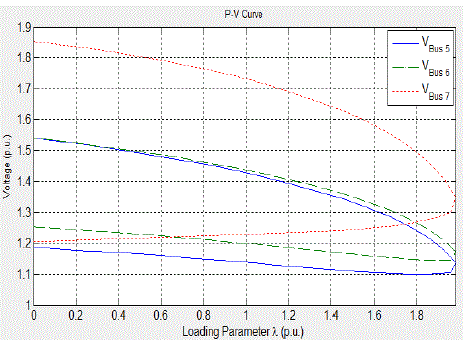 |
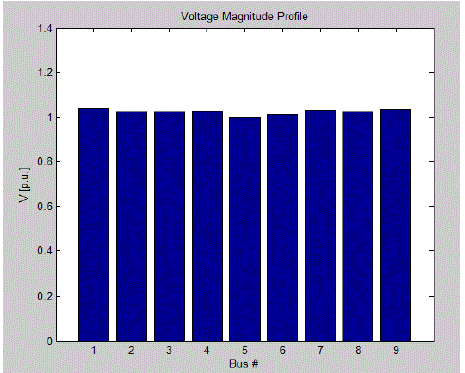 |
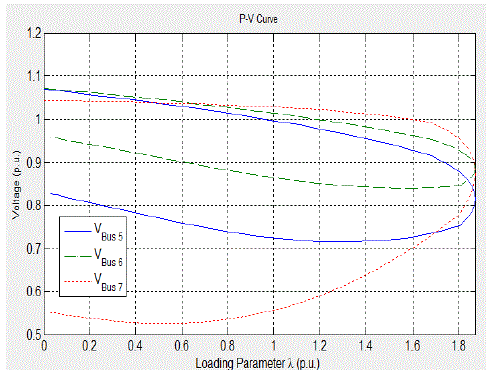 |
Figure 4b |
Figure 5a |
Figure 5b |
|
References
|
- P. Kundur, Power System Stability and Control, McGraw-Hill Inc., 1994.
- N. G. Hingorani and L. Gyugyi, “Understanding FACTS-concepts and technology of flexible AC transmission systems,” IEEE press, FirstIndian Edition, 2001.
- K. Kuthadi, N. Suresh, “Enhancement of Voltage Stability through Optimal Placement of Facts Controllers in Power Systems” AmericanJournal of Sustainable Cities and Society, vol. 1,July 2012.
- R. A. Schlueter, “A Voltage Stability Security Assessment Method,” IEEE Trans. On Power Systems, Vol.13, No.4, pp. 1423-1434, Nov.1998.
- F. A. Althowibi and M.W.Mustafa, “Voltage Stability Calculations in Power Transmission Lines: Indications and Allocations,” IEEE
- International Conference on Power and Energy, pp. 390-395, Nov.2010.
- ShravanaMusunuri, G.Dehnavi, “Comparison STATCOM, SVC, SSSC and TCSC devices for voltage stability improvement.”North AmericanPower Symposium (NAPS-2010), 26-28 Sept 2010
- Bhavin. M.Patel, “Enhancement of steady state voltage stability using SVC and TCSC” National Conference on Recent Trends in Engineeringand Technology, BVM Engineering College, Gujarat, India,13-14 May 2011.
- C. A. Canlzares, Z. T. Faur, "Analysis SVC and TCSC Controllers in Voltage Collapse," IEEE Trans. Power Systems, Vol. 14, No. I, pp.158-165, February 1999.
- P.Kessel and H.Glavitsch, “Estimating the Voltage Stability of a Power System” IEEE Transactions on Power Delivery, Vol. 1, No. 3, pp.346–354, 1986
- Mehrdad Ahmad, MostafaAlinezhad, “Comparison of SVC and STATCOM in static voltage stability margin enhancement”, Proceedingsof World Academy of Science, Engineering and Technology, Vol. 38, Feb. 2009.
- F. Milano, "Power System Analysis Toolbox," Version 1.3.4, Software and Documentation, July 14, 2005.
|